Nuclear medicine: PET, PET/CT, SPECT, SPECT/CT
Nuclear Medicine is the field dedicated to the imaging of short-lived radiolabelled compounds injected into the body, and is thus true ‘molecular imaging’. Due to its exquisite sensitivity, very low amounts of compounds that bind to cellular targets (radioligands) or are substrates for biological processes (radiotracers) can be detected, providing quantitative information about the rate of biological processes or number of receptors within organs and tissues without altering the underlying biology (the ‘tracer’ principle). Nuclear medicine makes use of two major modalities depending on the type of radioisotope used for radiolabelling, namely PET (Positron Emission Tomography) and SPECT (Single Photon Emission Computed Tomography).
PET and SPECT imaging can be performed as single modality acquisition but are more often used in combination with CT (PET-CT, SPECT-CT) or PET-MRI, which allow for precise spatial localization of the PET/SPECT signals within the body.
In PET (Positron Emission Tomography), radioligands and radiotracers contain isotopes which emit positrons upon nuclear decay. These positrons then annihilate in tissue to produce two nearly coincident high energy photons, which can be detected by a ring of detectors around the object (the PET scanner). Because both photons from an event are detected, PET uses electronic collimation and is thus more sensitive than SPECT (see below).
In microPET, the instrumentation is optimized for small animal studies and is thus usually of higher resolution.
Due to the low spatial resolution of PET images, PET is nowadays usually coupled to CT, or MRI, which afford higher resolution images for coregistration to provide spatial localization.
The most common PET tracer is [18F]-FDG, an analog of glucose that is taken up into cells and phosphorylated in the same way as glucose, but cannot undergo any of the subsequent steps of glycolysis. As such, it accumulates in tissues proportionally to the rate of glycolysis and is mainly used in clinics for the detection of cancer throughout the body due to the Warburg effect; it can also be used in clinical trials to detect early effects of cancer therapy. [18F]-FDG is also used to measure glycolysis in the brain, and is a biomarker of several neurodegenerative diseases.
Preclinically [18F]-FDG is also used in rodents for measuring brain and tumour glycolysis in disease models and in response to therapy.
In addition to [18F]-FDG, a wide range of other molecules have been radiolabelled to create radiotracers or radioligands using other radionuclides (e.g. Ga-68, C-11, O-18): these differ in radioactive half-life as well as the energy of the emitted positron. Typically small therapeutic molecules are labelled with C-11 and F-18 whilst larger biomolecules are labelled with Ga-68, Cu-64 and Zr-89. O-18 is used to radiolabel water for perfusion assessment.
Major PET applications revolve around the determination of receptor densities or metabolic reaction rates for a wide number of receptors and biological processes as detailed in the radiotracers table (T. Jones & P. Price, “Development and experimental medicine applications of PET in oncology: a historical perspective”, https://doi.org/10.1016/s1470-2045(11)70183-8; T. Jones, E.A. Rabiner, “The development, past achievements, and future directions of brain PET”, https://doi.org/10.1038/jcbfm.2012.20 ).
Importantly, radioligands can be used to assess the drug occupancy of therapeutics that bind to the same target as the PET tracer, which provides unique PK/PD information, especially in the brain (P.R. Matthews et al., “Positron emission tomography molecular imaging for drug development”, https://doi.org/10.1111/j.1365-2125.2011.04085.x).
In addition, longer-lived isotopes can be used to radiolabel a wide range of biotherapeutic compounds to allow the determination of biodistribution and tumour targeting in preclinical systems or in man (W. Wei et al., “ImmunoPET: Concept, Design, and Applications”, https://doi.org/10.1021/acs.chemrev.9b00738).
Finally, several PET tracers have been developed that are trapped by exogenously introduced genes, providing a reporter gene / theranostic imaging system that can be translated to man (C. Kitzberger et al., “The sodium iodide symporter (NIS) as theranostic gene: its emerging role in new imaging modalities and non-viral gene therapy”, https://doi.org/10.1186/s13550-022-00888-w).
Although PET is mostly widely used clinically and pre-clinically, it is also a powerful imaging tool for plant biologists. In fact, while current laboratory methods for the measurement of metabolic and transport processes in plants are destructive and allow monitoring only a very limited number of plants or sections of plants, PET allows to visualize non-invasively the metabolic and transport processes in the plant vascular systems and organs (S. Komarov & Y.C. Tai, “Positron Emission Tomography (PET) for Molecular Plant Imaging”, https://doi.org/10.1007/978-1-0716-2537-8_11).
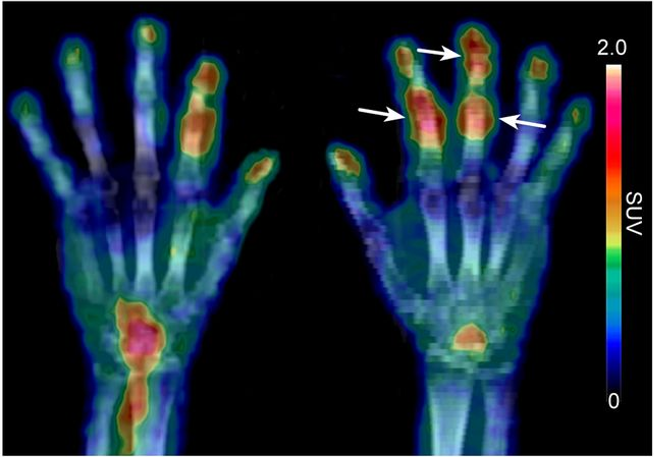
PET/CT
Due to the relatively low resolution of PET, it is usually combined with a CT scanner, with PET-CT images acquired sequentially (in the same session) and co-registered to allow the PET signal to be localized. The CT data is also used to correct the PET image for attenuation.
PET radioisotopes, tracers and related example applications from the Nodes
To view the PET radioisotopes available, click here. Please contact info@eurobioimaging.eu if you cannot find what you are looking for on our website.
As for PET, SPECT (Single Photon Emission Computed Tomography) radioligands and radiotracers contain radioisotopes. Unlike PET, these isotopes emit high energy photons that require physical collimation before detection by the SPECT scanner. It is thus less sensitive than PET.
The most commonly used radioisotope is Tc-99m, but a wide range of other radionuclides are also used, (e.g. In-111, I-123) that differ in the energy spectrum of the emitted photons. Although they also differ in radioactive half-life they are generally longer-lived than PET isotopes. Again, a wide range of molecules can thus be radiolabelled, though the requirement for a chelator prevents their use for small molecules.
In microSPECT, the instrumentation is optimized for small animal studies and is thus usually of higher resolution than either clinical SPECT or even preclinical PET, albeit with less sensitivity.
SPECT/CT
As for PET, CT can be combined with SPECT to allow for attenuation correction and to provide anatomical localization of the SPECT signal.
Read more on preclinical SPECT and SPECT/CT: B.L. Frank et al, “Preclinical SPECT and SPECT/CT in Oncology”, https://doi.org/10.1007/978-3-030-42618-7_11SPECT radioisotopes, tracers and related example applications from the Nodes
To view the SPECT radioisotopes available, click here.
Please contact info@eurobioimaging.eu if you cannot find what you are looking for on our website.
Nuclear Medicine images can be quantified in a number of ways, from qualitative evaluation to full quantitation of biologically relevant parameters (this latter is one of the unique aspects of Nuclear Imaging).
Qualitative
Qualitative evaluation is most often used in oncology, when the number (and location) of lesions provides valuable information. Qualitative imaging can be performed at any center.
Semiquantitative (SUV)
Semiquantitative imaging most often refers to the use of the Standardised Uptake Value (SUV), representing the tracer activity per unit volume normalised for injected dose and bodyweight. For many radiopharmaceuticals, SUV has been shown to be equivalent to fully quantitative approaches with the advantage of shorter scan times. However, it still depends on a number of experimental variables (e.g. type of anaesthesia and temperature control in animals, length of uptake period in animals and man). Semiquantitative imaging can be performed at most centers.
Fully Quantitative
Fully quantitative PET imaging requires the timecourse of parent (non-metabolised) radiotracer activity in blood to be collected alongside a dynamic PET scan of the organ(s) of interest; this is known as an ‘input function’. The input function can be convolved with various compartmental models to fit the dynamic PET data, the parameters of these models can be related to meaningful biological properties such as flux through a pathway or receptor density. Because of the need to sample blood and correct for metabolites, fully quantitative PET is usually available only at major PET centers throughout the world; preclinical studies are challenging due to the difficulty of obtaining enough blood at each timepoint.
REFERENCES.
A. Bertoldo et al, “Deriving physiological information from PET images: from SUV to compartmental modelling”, https://doi.org/10.1007/s40336...
A.A. Lammerstman, “Forward to the Past: The Case for Quantitative PET Imaging”, https://doi.org/10.2967/jnumed.116.188029
S.R. Meikle et al., “Quantitative PET in the 2020s: a Roadmap”, https://doi.org/10.1088/1361-6560/abd4f7
P. Ritt, “Recent Developments in SPECT/CT”, https://doi.org/10.1053/j.semnuclmed.2022.01.004
Preclinical PET and PET/CT are provided by the following Nodes:
- Austrian BioImaging / CMI (AT)
- Brain Imaging Network (PT)
- Center for Advanced Preclinical Imaging (CAPI) (CZ)
- Danish BioImaging (DK)
- DIMP Neuromed (IT) (also providing plant imaging)
- Facility of Multimodal Imaging - AMMI Maastricht (NL)
- Finnish Biomedical Imaging Node (FI)
- Flanders BioImaging Node (BE)
- Israel BioImaging (IL)
- Medical and Preclinical Imaging Hungary (HU)
- Molecular Imaging Italian Node (IT)
- NORMOLIM (NO)
- Preclinical Imaging Centre (PRIME)
- Population Imaging Node Valencia (ES)
- Swedish NMI (SE)
Human PET and PET/CT are provided by the following Nodes:
- Brain Imaging Network (PT)
- Finnish Biomedical Imaging Node (FI)
- Flanders BioImaging Node (BE)
- Medical and Preclinical Imaging Hungary (HU)
- Molecular Imaging Italian Node (IT)